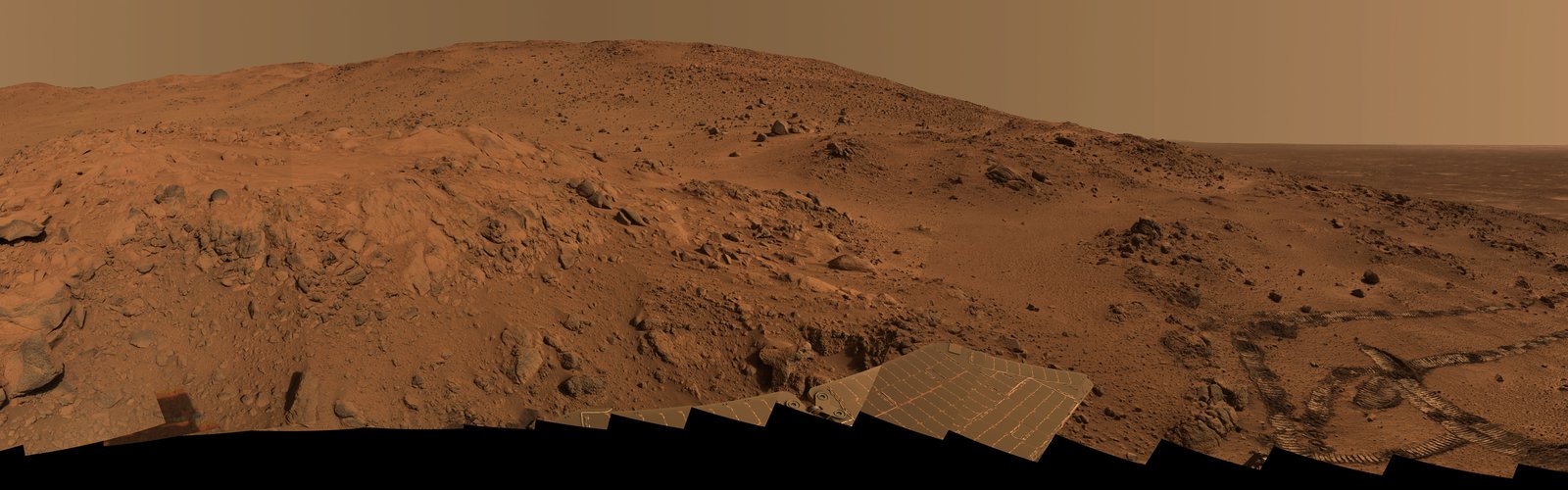
Geobiology Research Option
Planetary Geobiology
This exciting new discipline is a natural outgrowth of Caltech's strong traditions in geobiology, geochemistry, geology, and planetary science. If geobiology asks what the interactions are between life and environment on Earth, then planetary geobiology extends that question to other planetary bodies. There we can ask if the current or past history of our solar system's planets and moons are, or were ever, habitable for microbial life. Loosely defined, a habitable environment is one that has water, sources of carbon and nutrients to enable organism metabolism, and a source of energy to fuel organism metabolism – in other words, the essential ingredients for life as we know it on Earth. Furthermore, if microbial life did originate on those planets, then which environments and materials might best be sampled to test that hypothesis? This requires application of conceptual models for microbial and microfossil/biomarker exploration built on experience with Earth's geobiological record, global to local mapping by orbiting satellites, and landed missions using rovers equipped with advanced analytical laboratories. Increasingly, this research this seems a lot like doing geobiology on Earth, but with robots instead.
We live in the golden era of Mars exploration. Two rovers – Curiosity and Opportunity, and four orbiters – Mars Odyssey, Mars Express, Mars Reconnaissance Orbiter, and MAVEN routinely analyze the current and past states of the planet's environment. Each of these current missions has functioned so capably, performing well past their nominal operational periods, often with spectacular results and informing the broad range of environmental processes that transformed the surface of Mars, beginning over 4 billion years ago. Since landing at Gale crater in 2012 the Curiosity rover has driven over 15 km, studying rock formations dating back to 3.5 Ga, and thus overlapping with Earth's earliest well-preserved geobiological rock record — and enabling, for the first time, their direct comparison (see images below). Curiosity has returned a massive dataset to Earth that features results from drilling 18 unique samples of rock and soil involving dozens of laboratory analyses including x-ray diffraction, mass spectrometry, and gas chromatography; hundreds of rock samples have been analyzed via x-ray emission and laser-generated plasma emission spectra; thousands of images from the rover's seventeen cameras have been observed, along with millions of measurements of the atmosphere and radiation environment. All together, these data total over 15 Terabytes providing the basis for serious science for years to come.
Looking toward future missions of geobiological interest our faculty in the GPS division will be involved the next rover mission to Mars, to be launched in 2020, and beginning the process of collecting and analyzing samples for eventual return to Earth. In addition, we'll be involved in helping to develop the science rationale for the Europa Clipper mission which will explore that long sought icy moon of Jupiter, which harbors an alien sub-ice ocean.
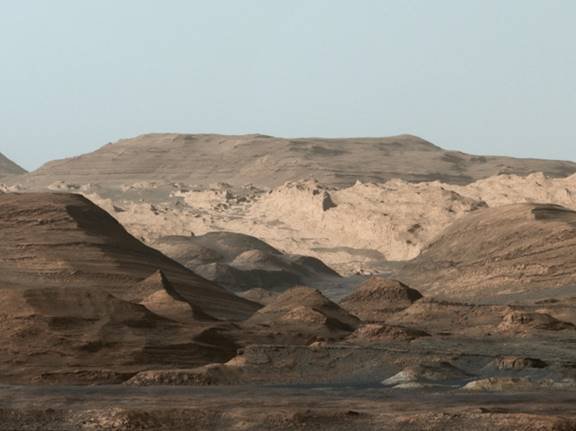
Image 1: The stratigraphic record of Gale Crater, Mars. These rocks are ~3.5 Ga and thus approximately similar in age to the oldest well-preserved stratigraphic record of Earth, in which we see the first direct microfossil evidence for life. This image is part of a Mastcam mosaic acquired by the Curiosity rover.
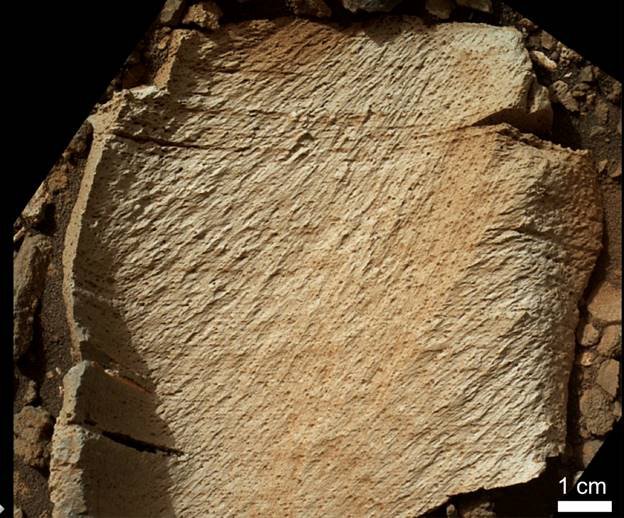
Image #2. The Murray formation at Gale crater, Mars, near Marias Pass exposes significant thicknesses of finely-laminated, fine-grained sedimentary rocks formed in an ancient lake system (Grotzinger et al., 2015) that is considered an ancient habitable environment (Grotzinger et al., 2014). This rock is composed of ~80% by weight crystalline silica in addition to significant amorphous silica such as Opal-A (Morris et al. 2016). The rock was formed by accumulation of siliceous sediments in a shallow lake, and as such is remarkably similar to certain types of sedimentary rocks on earth (see below).
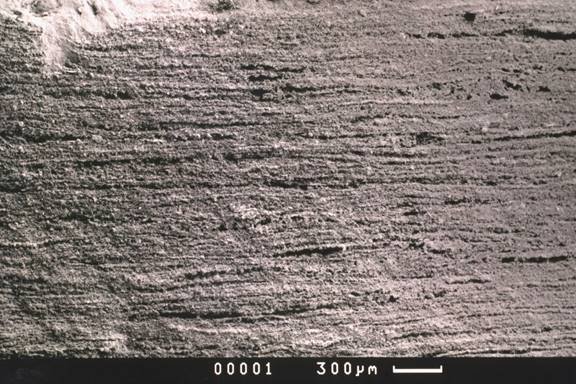
Image #3. The Athel "silicilyte", Precambrian-Cambrian boundary, Sultanate of Oman. This rocks is composed of up to ~80% by weight finely-laminated silica, formed by likely microbial or inorganic processes that precipitated silica at the Precambrian-Cambrian boundary (Ramseyer et al., 2013;)
References
Grotzinger, J. (and 72 others), 2014, A habitable fluvial-lacustrine environment at Yellowknife Bay, Gale crater, Mars. Science, 349. DOI: 10.1126/science.1242777.
Grotzinger J., (and 47 others), 2015, Deposition, exhumation, paleoclimate of an ancient lake deposit, Gale crater, Mars. Science, 350. DOI: 10.1126/science.aac7575.
Morris, R., (and 22 others), 2016, Silicic volcanism on Mars evidenced by tridymite detection in high-SiO2 sedimentary rock at gale crater.
Ramseyer, K., Amthor, J., Matter, A., Pettke, T., Wille, M., Fallick, A., 2012, Primary silica precipitate at the Precambrian/Cambrian boundary in the South Oman Salt Basin, Sultanate of Oman. Marine and Petroleum Geology, 39, 187-197.
Stolper, D., Love, G., Lyons, T., Young, E., Sessions, A., and Grotzinger, J., In Press, Paleoecology and paleoceanography of the Athel Silicilyte, Ediacaran-Cambrian boundary, Sultanate of Oman, Geobiology.