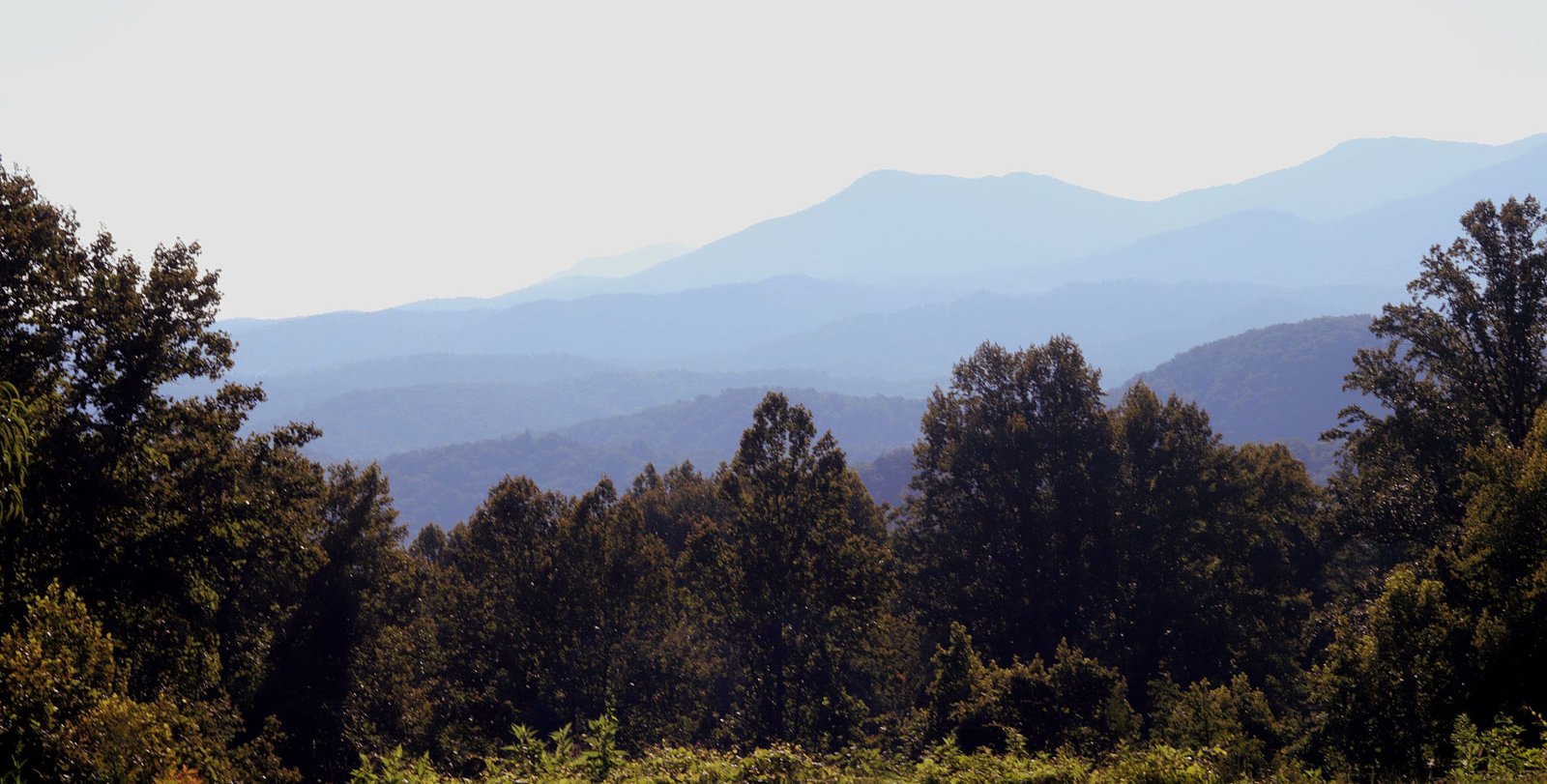
Chemists Tackle Formation of Natural Aerosols
City dwellers have long had to contend with smog—that ugly haze that hangs over urban areas—as a result of emissions-producing human activities as diverse as manufacturing, mowing the lawn, driving cars, and even cooking.
These emissions consist of gases such as carbon dioxide, nitrogen oxides, and volatile organic compounds (VOCs), as well as tiny solid particles known as aerosols. The haze you see when you look toward the horizon on a smoggy day is primarily these aerosol particles, which are both directly emitted to the atmosphere (and thus "primary aerosols") and also formed in the atmosphere ("secondary aerosols") due to the interaction of sunlight with compounds in the emissions, such as the VOCs.
Human activities are not the only source of aerosol particles, however. Trees and other vegetation also release VOCs that produce secondary aerosols through sunlight-driven chemistry, and in very large amounts. It is these aerosols, for example, that are responsible for the blue smokiness of the Great Smoky Mountains. Like their human-made counterparts, these natural aerosols affect air quality and also have significant impacts on the climate.
A new study conducted by researchers at Caltech is revealing for the first time key details about how the VOCs released by trees are transformed into aerosols through atmospheric chemistry. The paper describing the research, which appears in the journal Science, was a collaborative effort among the labs of John Seinfeld, the Louis E. Nohl Professor of Chemical Engineering; Paul Wennberg, the R. Stanton Avery Professor of Atmospheric Chemistry and Environmental Science and Engineering; and Brian Stoltz, the Victor and Elizabeth Atkins Professor of Chemistry and Heritage Medical Research Institute investigator.
"Somewhat counterintuitively, most aerosols in the global atmosphere do not come directly from human sources, and that is mainly just a product of the fact that forests make up a much larger fraction of the land surface than cities," says Christopher Kenseth (PhD '22), lead author of the paper and former Caltech chemistry graduate student, now a National Science Foundation (NSF) Postdoctoral Fellow at the University of Washington. "VOC emissions from plants and trees produce a substantial fraction of atmospheric aerosols globally, which play a pivotal role in the climate system."
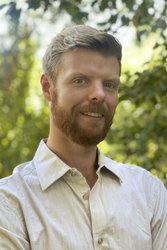
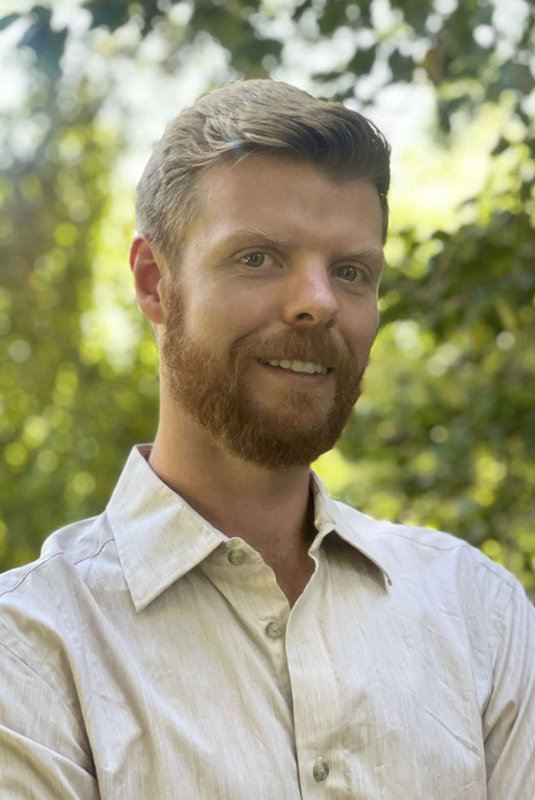
Kenseth says aerosols affect the climate in two ways: First, they reflect incoming sunlight, preventing it from reaching Earth's surface (just like they might block your view of the mountains on a smoggy day in Los Angeles). Second, they act as a seed for the formation of clouds, which also reflect sunlight back into space. In fact, without aerosol particles, there would be many fewer clouds in the atmosphere.
Plants and trees emit countless compounds that form secondary aerosols, but in this study the researchers focused specifically on a pair of compounds called alpha-pinene and beta-pinene, which are emitted from conifers and give the trees their characteristic piney smell. These pinenes constitute a major portion of the VOCs released in forested areas and are consequently responsible for much of the aerosol formation.
The importance of the global formation of atmospheric aerosols has been known for decades, and the so-called "pinene system" has been studied for more than 40 years. In the past two decades, multiple analyses have shown that dimers (compounds made up of two smaller molecules that are joined together by a chemical bond) are major components of pinene-derived aerosol. However, because the oxidation chemistry that forms aerosol from pinene is extremely complex, atmospheric chemists previously had developed only educated guesses as to the identities of these dimers and, by extension, how they form.
In the current study, Kenseth relied on resources in the Seinfeld, Wennberg, and Stoltz labs to uncover the structures and formation mechanism of dimers identified in pinene-derived aerosol using a combination of laboratory experiments and organic synthesis.
"Given the recognized importance of pinene dimers, it is surprising that the mechanism for their formation had remained opaque for so long," Wennberg says. "It is really a tribute to the ability to synthesize the putative compounds and study their behavior that enabled this science."
Kenseth generated pinene-derived aerosol in the Caltech Environmental Chamber, a large (24,000 liter) Teflon bag that simulates the real atmosphere but allows tight control of conditions such as temperature and humidity. By collecting the aerosol on filters and analyzing its molecular composition using mass spectrometry, Kenseth was able to propose structures for the major dimers identified in the aerosol samples. Kenseth then collaborated with researchers in the Stoltz lab to synthesize the proposed compounds and then determined, again using mass spectrometry, that the structures of the synthesized dimers matched those of the dimers identified in the aerosol.
"It was something that we got excited about," Stoltz says. "The things we usually work on are super complicated. These aerosol compounds are very tiny molecules in comparison but have their own complexities."
Having definitively confirmed the structures of the dimers, Kenseth carried out additional experiments in the Caltech chamber to decipher the detailed chemical mechanism by which they form. Critically, the experiments showed that the bond linking the two halves of the dimer is formed in the aerosol particles, as opposed to when the oxidation products are present as gases. "This resolved a long-standing puzzle in aerosol chemistry," says Kenseth. "We have known for decades that these dimers are important drivers of aerosol production, but only by synthesizing authentic standards were we able to concretely determine their structures and then design the experiments that unraveled their formation mechanism."
This discovery is important to atmospheric chemists like Seinfeld and Wennberg because it fills a key gap in the field's understanding of the composition and formation chemistry of atmospheric aerosols, knowledge that is essential for an accurate assessment of their environmental and health impacts.
"Knowing how they form allows us to understand what other compounds may also produce such aerosols. Without a mechanism, we would need to search through the entire catalog of VOCs—something that would be essentially impossible," Wennberg says.
Seinfeld adds: "Characterizing the molecular-level details of the chemistry of aerosol formation is arguably the most challenging research area in atmospheric chemistry. This study is a landmark not only in terms of the methodology employed, but because it represents a rare case in this field wherein all aspects of an important complex chemical reaction are now well understood."
The paper describing the research, "Particle-Phase Accretion Forms Dimer Esters in Pinene Secondary Organic Aerosol," appears in the November 17 issue of Science. Co-authors are Nicholas Hafeman (PhD '22), of AbbVie Inc.; Samir Rezgui, Caltech graduate student in chemistry; Jing Chen of the University of Copenhagen; Yuanlong Huang (PhD '19) of the Eastern Institute for Advanced Study; Nathan Dalleska, director of the Resnick Water and Environment Lab at Caltech; Henrik Kjaergaard of the University of Copenhagen; as well as Seinfeld, Wennberg, and Stoltz.
Funding for the research was provided by the NSF, the Independent Research Fund Denmark, the Villum Foundation, and the Alfred P. Sloan Foundation.